Antimatter atoms yield high-precision measurement
If antihydrogen has an electric charge, it's too small to measure — so far
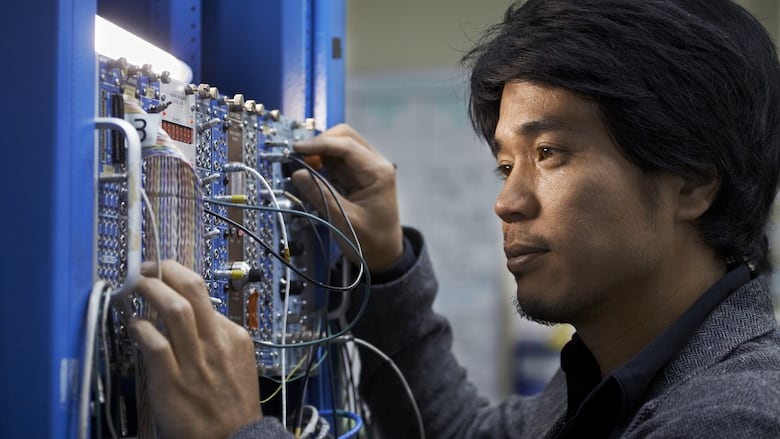
Scientists have conducted an extremely precise measurement of the charge of antimatter atoms, bringing them a step closer to figuring out one of the great mysteries of physics — why our universe is almost entirely made of matter.
The ALPHA collaboration, an international group of scientists including many Canadians, has now measured the charge of antihydrogen — the antimatter version of hydrogen — and found that it is neutral or zero to eight decimal places. That is, if it has a charge, the biggest it could be and still be undetectable is 0.00000001 times or a hundred-millionth the charge of electron.
"This represents the first precision measurement of antimatter atom's properties," said Makoto Fujiwara, who leads the Canadian branch of the ALPHA team and played a key role in the achievement, described Tuesday in Nature Communications.
Previously, Fujiwara said, scientists had been focused on developing technology and techniques to study antihydrogen, such as techniques to trap them.
"This is the first measurement that allows us to improve the knowledge of the fundamental properties of antimatter."
Antimatter is made up of "antiparticles" that have the same mass as corresponding particles of matter, but an opposite charge. For example, the antimatter counterpart of a negatively charged electron is a positively charged positron.
Antimatter is very difficult to keep in existence for more than tiny fractions of seconds, because the moment it touches matter, which makes up most of our universe, both the matter and antimatter are annihilated, producing pure energy.
In 2010, the ALPHA collaboration developed a technique that allows it to trap antihydrogen for long periods of time. In 2011, it announced it could keep antimatter in existence for up to 16 minutes.
Scientists are interested in studying antimatter in order to understand why our universe is mostly matter, even though antimatter and matter should be made in equal amounts when energy is converted into mass, as is thought to have happened during the Big Bang.
"Everything we do on antihydrogen is related to trying to see some subtle difference between matter and antimatter," said Scott Menary, a York University researcher who also played a major role in the discovery.
"There obviously had to have been some subtle effect, which at some point in the universe just lopped over a little bit on the matter side, and then all of the rest of the matter and antimatter annihilated so we're just basically left with matter."
In the case of charge, so far there is no detectable difference between hydrogen and antihydrogen.
Of course, it was expected that antihydrogen would be neutral, said Fujiwara, a research scientist at Vancouver-based TRIUMF, Canada's national laboratory for particle and accelerator physics. According to basic laws of physics, the magnitudes of the charges of matter and antimatter should be the same (although they should have the opposite sign).
"So if you see any difference — even at parts per trillion — then that would mean that basic laws of physics have to be modified."
Fujiwara cautioned, though, that there may still be a difference too small to detect, and therefore more experiments need to be conducted.
Measurement not originally planned
Interestingly, the researchers had never intended to measure the charge of an antihydrogen, but were conducting other experiments to improve techniques to trap antimatter.
At the end of the experiment, the antimatter was released, hit the matter on the side of the container, and was annihilated.
Scientists needed to rule out the possibility that the annhilations were caused by antiprotons rather than antihydrogen atoms. Antiprotons have a charge of "-1" — equal to that of an electron — so they respond to electric fields, unlike antihydrogen atoms, which are expected to be neutral. To rule out the presence of antiprotons, before measuring annihilation, the researchers applied an electric field to sweep away any antiprotons that might be there.
Fujiwara realized that if the antihydrogen atoms had even a slight charge, they would be influenced subtly by the electric field too. He wondered if the data already collected over two summers would show antihydrogen atoms pushed more in one direction to another by the electric field.
Menary and his students analyzed the data to determine where the antihydrogen atoms annihilated with respect to the electric field. They also did a painstaking analysis to ensure that the results were not influenced by subtle differences in the experimental setup during the two different summers. In the end, the team confirmed that there were no differences and the antihydrogen atoms did not respond at all to the electric field.
The ALPHA team has recently built a new experimental apparatus at the antiproton decelerator run by CERN, the European Organization for Nuclear Research, near the French-Swiss border, where the team conducts its experiments. That will allow the ALPHA team to take new and more precise measurements. The apparatus, which had many core parts developed and built in Canada, will let scientists shoot laser beams into antimatter atoms for the first time, and also drop antimatter atoms to see if they fall the same way as matter, Fujiwara said.
In addition to Fujiwara, Menary and their research teams, the ALPHA collaboration includes scientists from the University of British Columbia, Simon Fraser University and the University of Calgary, along with scientists in Brazil, Denmark, Israel, Japan, Sweden, the U.K. and the U.S.